- This is a discussion of a present category of science. For the work by Aristotle, see Physics (Aristotle). For a history of the science, see History of physics. For the etymology of the word "physics," see physis (φύσις).
Physics is the science of matter[1] and its motion,[2][3] as well as space and time.[4][5] It uses concepts such as energy, force, mass, and charge. Physics is an experimental science,[6] creating theories that are tested against observations. Broadly, it is the general scientific analysis of nature, with a goal of understanding how the universe behaves.[7]
Physics is one of the oldest academic disciplines, and through its modern subfield of astronomy, it may be the oldest of all.[8] Experimental physics began in the Middle Ages and eventually emerged as a modern science during the early modern period.[9] Those who work professionally in the field are known as physicists.
Advances in physics often translate to the technological sector, and often influence the other sciences, as well as mathematics and philosophy. For example, advances in the understanding of electromagnetism have led to the widespread use of electrically driven devices (televisions, computers, home appliances etc.); advances in thermodynamics led to the development of motorized transport; and advances in mechanics motivated and benefitted from the development of calculus, quantum chemistry, and the use of instruments such as the electron microscope in microbiology. The "Atomic Age" is also an important concept in philosophy and historical analysis.
Today, physics is a broad and highly developed subject. Research is often divided into four subfields: condensed matter physics; atomic, molecular, and optical physics; high-energy physics; and astronomy and astrophysics. Most physicists also specialize in either theoretical or experimental research, the former dealing with the development of new theories, and the latter dealing with the experimental testing of theories and the discovery of new phenomena. Despite important discoveries during the last four centuries, there are a number of unsolved problems in physics, and many areas of active research.
Contents[hide] |
[edit] Branches of physics
Although physics encompasses a wide variety of phenomena, the fundamental branches of physics are classical mechanics, electromagnetism (including optics), relativity, thermodynamics, and quantum mechanics. Each of these theories has been tested in numerous experiments and proven to be an accurate model of nature within its domain of validity. For example, classical mechanics correctly describes the motion of objects in everyday experience, but it breaks down at the atomic scale, where it is superseded by quantum mechanics, and at speeds approaching the speed of light, where relativistic effects become important. While these theories have long been well-understood, they continue to be areas of active research — for example, a remarkable aspect of classical mechanics known as chaos theory was developed in the 20th century, three centuries after the original formulation of mechanics by Isaac Newton (1642–1727). The basic theories form a foundation for the study and research of more specialized topics.
A table of these theories, along with many of the concepts they employ, is available.
[edit] Classical mechanics

Classical mechanics is a model of the physics of forces acting upon bodies. It is often referred to as "Newtonian mechanics" after Isaac Newton and his laws of motion. Mechanics is subdivided into statics, which models objects at rest, kinematics, which models objects in motion, and dynamics, which models objects subjected to forces. The classical mechanics of continuous and deformable objects is continuum mechanics, which can itself be broken down into solid mechanics and fluid mechanics according to the state of matter being studied. The latter, the mechanics of liquids and gases, includes hydrostatics, hydrodynamics, pneumatics, aerodynamics, and other fields. Mechanical Statics deals with objects at rest. Mechanical kinematics deals with objects in motion. Mechanical dynamics deals with motion of objects subject to forces.
Classical mechanics produces accurate results within the domain of everyday experience. It is superseded by relativistic mechanics for systems moving at large velocities near the speed of light, quantum mechanics for systems at small distance scales, and relativistic quantum field theory for systems with both properties. Nevertheless, classical mechanics is still useful, because it is much simpler and easier to apply than these other theories, and it has a very large range of approximate validity. Classical mechanics can be used to describe the motion of human-sized objects (such as tops and baseballs), many astronomical objects (such as planets and galaxies), and certain microscopic objects (such as organic molecules).
An important concept of mechanics is the identification of conserved energy and momentum, which lead to the Lagrangian and Hamiltonian reformulations of Newton's laws. Theories such as fluid mechanics and the kinetic theory of gases result from applying classical mechanics to macroscopic systems. A relatively recent result of considerations concerning the dynamics of nonlinear systems is chaos theory, the study of systems in which small changes in a variable may have large effects. Newton's law of universal gravitation, formulated within classical mechanics, explained Kepler's laws of planetary motion and helped make classical mechanics an important element of the Scientific Revolution.
[edit] Electromagnetism
- See also: Optics
Electromagnetism describes the interaction of charged particles with electric and magnetic fields. It can be divided into electrostatics, the study of interactions between charges at rest, and electrodynamics, the study of interactions between moving charges and radiation. The classical theory of electromagnetism is based on the Lorentz force law and Maxwell's equations.
Electrostatics is the study of phenomena associated with charged bodies at rest. As described by Coulomb’s law, such bodies exert forces on each other. Their behavior can be analyzed in terms of the concept of an electric field surrounding any charged body, such that another charged body placed within the field is subject to a force proportional to the magnitude of its own charge and the magnitude of the field at its location. Whether the force is attractive or repulsive depends on the polarity of the charge. Electrostatics has many applications, ranging from the analysis of phenomena such as thunderstorms to the study of the behavior of electron tubes.
Electrodynamics is the study of phenomena associated with charged bodies in motion and varying electric and magnetic fields. Since a moving charge produces a magnetic field, electrodynamics is concerned with effects such as magnetism, electromagnetic radiation, and electromagnetic induction, including such practical applications as the electric generator and the electric motor. This area of electrodynamics, known as classical electrodynamics, was first systematically explained by James Clerk Maxwell, and Maxwell’s equations describe the phenomena of this area with great generality. A more recent development is quantum electrodynamics, which incorporates the laws of quantum theory in order to explain the interaction of electromagnetic radiation with matter. Paul Dirac, Werner Heisenberg, and Wolfgang Pauli were pioneers in the formulation of quantum electrodynamics. Relativistic electrodynamics accounts for relativistic corrections to the motions of charged particles when their speeds approach the speed of light. It applies to phenomena involved with particle accelerators and electron tubes carrying high voltages and currents.
Electromagnetism encompasses various real-world electromagnetic phenomena. For example, light is an oscillating electromagnetic field that is radiated from accelerating charged particles. Aside from gravity, most of the forces in everyday experience are ultimately a result of electromagnetism.
The principles of electromagnetism find applications in various allied disciplines such as microwaves, antennas, electric machines, satellite communications, bioelectromagnetics, plasmas, nuclear research, fiber optics, electromagnetic interference and compatibility, electromechanical energy conversion, radar meteorology, and remote sensing. Electromagnetic devices include transformers, electric relays, radio/TV, telephones, electric motors, transmission lines, waveguides, optical fibers, and lasers.
[edit] Relativity
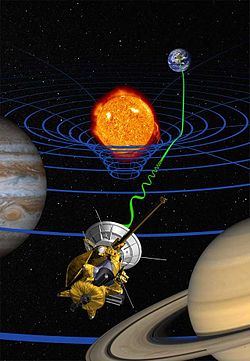
Relativity is a generalization of classical mechanics that describes fast-moving or very massive systems. It includes special and general relativity.
The theory of special relativity was proposed in 1905 by Albert Einstein in his article "On the Electrodynamics of Moving Bodies". The title of the article refers to the fact that special relativity resolves an inconsistency between Maxwell's equations and classical mechanics. The theory is based on two postulates: (1) that the mathematical forms of the laws of physics are invariant in all inertial systems; and (2) that the speed of light in a vacuum is constant and independent of the source or observer. Reconciling the two postulates requires a unification of space and time into the frame-dependent concept of spacetime.
Special relativity has a variety of surprising consequences that seem to violate common sense, but all have been experimentally verified. It overthrows Newtonian notions of absolute space and time by stating that distance and time depend on the observer, and that time and space are perceived differently, depending on the observer. The theory leads to the assertion of change in mass, dimension, and time with increased velocity. It also yields the equivalence of matter and energy, as expressed in the mass-energy equivalence formula E = mc2, where c is the speed of light in a vacuum. Special relativity and the Galilean relativity of Newtonian mechanics agree when velocities are small compared to the speed of light. Special relativity does not describe gravitation; however, it can handle accelerated motion in the absence of gravitation.[10]
General relativity is the geometrical theory of gravitation published by Albert Einstein in 1915/16.[11][12] It unifies special relativity, Newton's law of universal gravitation, and the insight that gravitation can be described by the curvature of space and time. In general relativity, the curvature of space-time is produced by the energy of matter and radiation. General relativity is distinguished from other metric theories of gravitation by its use of the Einstein field equations to relate space-time content and space-time curvature. Local Lorentz Invariance requires that the manifolds described in GR be 4-dimensional and Lorentzian instead of Riemannian. In addition, the principle of general covariance forces that mathematics be expressed using tensor calculus.
The first success of general relativity was in explaining the anomalous perihelion precession of Mercury. Then in 1919, Sir Arthur Eddington announced that observations of stars near the eclipsed Sun confirmed general relativity's prediction that massive objects bend light. Since then, many other observations and experiments have confirmed many of the predictions of general relativity, including gravitational time dilation, the gravitational redshift of light, signal delay, and gravitational radiation. In addition, numerous observations are interpreted as confirming one of general relativity's most mysterious and exotic predictions, the existence of black holes.
[edit] Thermodynamics and statistical mechanics

Thermodynamics studies the effects of changes in temperature, pressure, and volume on physical systems at the macroscopic scale, and the transfer of energy as heat.[13][14] Historically, thermodynamics developed out of need to increase the efficiency of early steam engines.[15]
The starting point for most thermodynamic considerations are the laws of thermodynamics, which postulate that energy can be exchanged between physical systems as heat or work.[16] They also postulate the existence of a quantity named entropy, which can be defined for any system.[17] In thermodynamics, interactions between large ensembles of objects are studied and categorized. Central to this are the concepts of system and surroundings. A system is composed of particles, whose average motions define its properties, which in turn are related to one another through equations of state. Properties can be combined to express internal energy and thermodynamic potentials, which are useful for determining conditions for equilibrium and spontaneous processes.
Statistical mechanics analyzes macroscopic systems by applying statistical principles to their microscopic constituents. It provides a framework for relating the microscopic properties of individual atoms and molecules to the macroscopic or bulk properties of materials that can be observed in everyday life. Thermodynamics can be explained as a natural result of statistics and mechanics (classical and quantum) at the microscopic level. In this way, the gas laws can be derived, from the assumption that a gas is a collection of individual particles, as hard spheres with mass. Conversely, if the individual particles are also considered to have charge, then the individual accelerations of those particles will cause the emission of light. It was these considerations which caused Max Planck to formulate his law of blackbody radiation,[18] but only with the assumption that the spectrum of radiation emitted from these particles is not continuous in frequency, but rather quantized.[19]
[edit] Quantum mechanics

Quantum mechanics is the branch of physics treating atomic and subatomic systems and their interaction with radiation in terms of observable quantities. It is based on the observation that all forms of energy are released in discrete units or bundles called "quanta". Remarkably, quantum theory typically permits only probable or statistical calculation of the observed features of subatomic particles, understood in terms of wavefunctions. The Schrödinger equation plays the role in quantum mechanics that Newton's laws and conservation of energy serve in classical mechanics — i.e., it predicts the future behavior of a dynamic system — and is a wave equation in terms of the wavefunction which predicts analytically and precisely the probability of events or outcomes.
According to the older theories of classical physics, energy is treated solely as a continuous phenomenon, while matter is assumed to occupy a specific region of space and to move in a continuous manner. According to the quantum theory, energy is held to be emitted and absorbed in tiny, discrete amounts. An individual bundle or packet of energy, called a quantum (pl. quanta), thus behaves in some situations much like particles of matter; particles are found to exhibit certain wavelike properties when in motion and are no longer viewed as localized in a given region but rather as spread out to some degree. For example, the light, or electromagnetic radiation, emitted or absorbed by an atom has only certain frequencies (or wavelengths), as can be seen from the line spectrum associated with the chemical element represented by that atom. The quantum theory shows that those frequencies correspond to definite energies of the light quanta, or photons, and result from the fact that the electrons of the atom can have only certain allowed energy values, or levels; when an electron changes from one allowed level to another, a quantum of energy is emitted or absorbed whose frequency is directly proportional to the energy difference between the two levels.
The formalism of quantum mechanics was developed during the 1920s. In 1924, Louis de Broglie proposed that not only do light waves sometimes exhibit particle-like properties, as in the photoelectric effect and atomic spectra, but particles may also exhibit wavelike properties. Two different formulations of quantum mechanics were presented following de Broglie’s suggestion. The wave mechanics of Erwin Schrödinger (1926) involves the use of a mathematical entity, the wave function, which is related to the probability of finding a particle at a given point in space. The matrix mechanics of Werner Heisenberg (1925) makes no mention of wave functions or similar concepts but was shown to be mathematically equivalent to Schrödinger’s theory. A particularly important discovery of the quantum theory is the uncertainty principle, enunciated by Heisenberg in 1927, which places an absolute theoretical limit on the accuracy of certain measurements; as a result, the assumption by earlier scientists that the physical state of a system could be measured exactly and used to predict future states had to be abandoned. Quantum mechanics was combined with the theory of relativity in the formulation of Paul Dirac (1928), which, in addition, predicted the existence of antiparticles. Other developments of the theory include quantum statistics, presented in one form by Einstein and S. N. Bose (the Bose-Einstein statistics) and in another by Dirac and Enrico Fermi (the Fermi-Dirac statistics); quantum electrodynamics, concerned with interactions between charged particles and electromagnetic fields; its generalization, quantum field theory; and quantum electronics. The discovery of quantum mechanics in the early 20th century revolutionized physics, and quantum mechanics is fundamental to most areas of current research.
[edit] Research
[edit] Theory and experiment
The culture of physics research differs from most sciences in the separation of theory and experiment. Since the twentieth century, most individual physicists have specialized in either theoretical physics or experimental physics. The great Italian physicist Enrico Fermi (1901–1954), who made fundamental contributions to both theory and experimentation in nuclear physics, was a notable exception. In contrast, almost all the successful theorists in biology and chemistry (e.g. American quantum chemist and biochemist Linus Pauling) have also been experimentalists, although this is changing as of late.
Theorists seek to develop mathematical models that both agree with existing experiments and successfully predict future results, while experimentalists devise and perform experiments to test theoretical predictions and explore new phenomena. Although theory and experiment are developed separately, they are strongly dependent upon each other. Progress in physics frequently comes about when experimentalists make a discovery that existing theories cannot explain, or when new theories generate experimentally testable predictions. Theorists working closely with experimentalists frequently employ phenomenology.
Theoretical physics is closely related to mathematics, which provides the language of physical theories, and large areas of mathematics, such as calculus, have been invented specifically to solve problems in physics. Theorists may also rely on numerical analysis and computer simulations, which play an ever richer role in the formulation of physical models. The fields of mathematical and computational physics are active areas of research. Theoretical physics has historically rested on philosophy and metaphysics; electromagnetism was unified this way.[20] Thus physicists may speculate with multidimensional spaces and parallel universes, and from this, hypothesize theories.
Experimental physics informs, and is informed by, engineering and technology. Experimental physicists involved in basic research design and perform experiments with equipment such as particle accelerators and lasers, whereas those involved in applied research often work in industry, developing technologies such as magnetic resonance imaging (MRI) and transistors. Feynman has noted that experimentalists may seek areas which are not well explored by theorists.
[edit] Research fields
Contemporary research in physics can be broadly divided into condensed matter physics; atomic, molecular, and optical physics; particle physics; astrophysics; geophysics and biophysics. Some physics departments also support research in Physics education. Since the twentieth century, the individual fields of physics have become increasingly specialized, and today most physicists work in a single field for their entire careers. "Universalists" such as Albert Einstein (1879–1955) and Lev Landau (1908–1968), who worked in multiple fields of physics, are now very rare.[21] A table of the major fields of physics, along with their subfields and the theories they employ, can be found here.
[edit] Condensed matter

Condensed matter physics is the field of physics that deals with the macroscopic physical properties of matter. In particular, it is concerned with the "condensed" phases that appear whenever the number of constituents in a system is extremely large and the interactions between the constituents are strong. The most familiar examples of condensed phases are solids and liquids, which arise from the bonding and electromagnetic force between atoms. More exotic condensed phases include the superfluid and the Bose-Einstein condensate found in certain atomic systems at very low temperature, the superconducting phase exhibited by conduction electrons in certain materials, and the ferromagnetic and antiferromagnetic phases of spins on atomic lattices.
Condensed matter physics is by far the largest field of contemporary physics. Much progress has also been made in theoretical condensed matter physics. By one estimate, one third of all American physicists identify themselves as condensed matter physicists. Historically, condensed matter physics grew out of solid-state physics, which is now considered one of its main subfields. The term condensed matter physics was apparently coined by Philip Anderson when he renamed his research group — previously solid-state theory — in 1967. In 1978, the Division of Solid State Physics at the American Physical Society was renamed as the Division of Condensed Matter Physics.[22] Condensed matter physics has a large overlap with chemistry, materials science, nanotechnology and engineering.
[edit] Atomic, molecular, and optical
Atomic, molecular, and optical physics (AMO) is the study of matter-matter and light-matter interactions on the scale of single atoms or structures containing a few atoms. The three areas are grouped together because of their interrelationships, the similarity of methods used, and the commonality of the energy scales that are relevant. All three areas include both classical and quantum treatments; they can treat their subject from a microscopic view (in contrast to a macroscopic view).
Atomic physics studies the electron hull of atoms. Current research focuses on activities in quantum control, cooling and trapping of atoms and ions, low-temperature collision dynamics, the collective behavior of atoms in weakly interacting gases (Bose-Einstein Condensates and dilute Fermi degenerate systems), precision measurements of fundamental constants, and the effects of electron correlation on structure and dynamics. Atomic physics is influenced by the nucleus (see, e.g., hyperfine splitting), but intra-nuclear phenomenon such as fission and fusion are considered part of high energy physics.
Molecular physics focuses on multi-atomic structures and their internal and external interactions with matter and light. Optical physics is distinct from optics in that it tends to focus not on the control of classical light fields by macroscopic objects, but on the fundamental properties of optical fields and their interactions with matter in the microscopic realm.
[edit] High energy/particle physics

Particle physics is the study of the elementary constituents of matter and energy, and the interactions between them. It may also be called "high energy physics", because many elementary particles do not occur naturally, but are created only during high energy collisions of other particles, as can be detected in particle accelerators.
Currently, the interactions of elementary particles are described by the Standard Model. The model accounts for the 12 known particles of matter that interact via the strong, weak, and electromagnetic fundamental forces. Dynamics are described in terms of matter particles exchanging messenger particles that carry the forces. These messenger particles are known as gluons, W− and W+ and Z bosons, and the photons, respectively. The Standard Model also predicts a particle known as the Higgs boson, the existence of which has not yet been verified.
[edit] Astrophysics
Astrophysics and astronomy are the application of the theories and methods of physics to the study of stellar structure, stellar evolution, the origin of the solar system, and related problems of cosmology. Because astrophysics is a broad subject, astrophysicists typically apply many disciplines of physics, including mechanics, electromagnetism, statistical mechanics, thermodynamics, quantum mechanics, relativity, nuclear and particle physics, and atomic and molecular physics.
Astrophysics developed from the ancient science of astronomy. Astronomers of early civilizations performed methodical observations of the night sky, and astronomical artifacts have been found from much earlier periods. After centuries of developments by Babylonian and Greek astronomers, western astronomy lay dormant for fourteen centuries until Nicolaus Copernicus modified the Ptolemaic system by placing the sun at the center of the universe. Tycho Brahe's detailed observations led to Kepler's laws of planetary motion, and Galileo's telescope helped the discipline develop into a modern science. Isaac Newton's theory of universal gravitation provided a physical, dynamic basis for Kepler's laws. By the early 19th cent., the science of celestial mechanics had reached a highly developed state at the hands of Leonhard Euler, J. L. Lagrange, P. S. Laplace, and others. Powerful new mathematical techniques allowed solution of most of the remaining problems in classical gravitational theory as applied to the solar system. At the end of the 19th century, the discovery of spectral lines in sunlight proved that the chemical elements found in the Sun were also found on Earth. Interest shifted from determining the positions and distances of stars to studying their physical composition (see stellar structure and stellar evolution). Because the application of physics to astronomy became increasingly important throughout the 20th century, the distinction between astronomy and astrophysics has faded.
The discovery by Karl Jansky in 1931 that radio signals were emitted by celestial bodies initiated the science of radio astronomy. Most recently, the frontiers of astronomy have been expanded by space exploration. Perturbations and interference from the earth’s atmosphere make space-based observations necessary for infrared, ultraviolet, gamma-ray, and X-ray astronomy. The Hubble Space Telescope, launched in 1990, has made possible visual observations of a quality far exceeding those of earthbound instruments; earth-bound observatories using telescopes with adaptive optics will now be able to compensate for the turbulence of Earth's atmosphere.
Physical cosmology is the study of the formation and evolution of the universe on its largest scales. Albert Einstein’s theory of relativity plays a central role in all modern cosmological theories. In the early 20th century, Hubble's discovery that the universe was expanding, as shown by the Hubble diagram, prompted rival explanations known as the steady state universe and the Big Bang. The Big Bang was confirmed by the success of Big Bang nucleosynthesis and the discovery of the cosmic microwave background in 1964. The Big Bang model rests on two theoretical pillars: Albert Einstein's general relativity and the cosmological principle. Cosmologists have recently established a precise model of the evolution of the universe, which includes cosmic inflation, dark energy and dark matter.
[edit] Applied physics
Applied physics is a general term for physics which is intended for a particular use. Applied is distinguished from pure by a subtle combination of factors such as the motivation and attitude of researchers and the nature of the relationship to the technology or science that may be affected by the work.[23] It usually differs from engineering in that an applied physicist may not be designing something in particular, but rather is using physics or conducting physics research with the aim of developing new technologies or solving a problem. The approach is similar to that of applied mathematics. Applied physicists can also be interested in the use of physics for scientific research. For instance, people working on accelerator physics might seek to build better particle detectors for research in theoretical physics.
Physics is used heavily in engineering. For example, statics, a subfield of mechanics, is used in the building of bridges or other structures, while acoustics is used to design better concert halls. An understanding of physics is important to the design of realistic flight simulators, video game physics engines, and movies.
[edit] Physics Education
Physics education refers both to the methods currently used to teach physics, and to an area of pedagogical research that seeks to improve those methods. Historically, physics has been taught at the high school and university level primarily by the lecture method, together with laboratory exercises aimed at verifying concepts taught in the lectures.
[edit] References
- ^ R. P. Feynman, R. B. Leighton, M. Sands (1963), The Feynman Lectures on Physics, ISBN 0-201-02116-1 Hard-cover. p.1-1 Feynman begins with the atomic hypothesis, as his most compact statement of all scientific knowledge: "If, in some cataclysm, all of scientific knowledge were to be destroyed, and only one sentence passed on to the next generations ..., what statement would contain the most information in the fewest words? I believe it is ... that all things are made up of atoms -- little particles that move around in perpetual motion, attracting each other when they are a little distance apart, but repelling upon being squeezed into one another. ..." vol. I p. I-2
- ^ James Clerk Maxwell (1876), Matter and Motion. Notes and appendices by Joseph Larmor. "Physical science is that department of knowledge which relates to the order of nature, or, in other words, to the regular succession of events". p.1
- ^ "Give me matter and motion, and I will construct the universe." --Rene Descartes (1596-1650)
- ^ Inquiring Minds
- ^ E.F. Taylor, J.A. Wheeler (2000), Exploring Black Holes: Introduction to General Relativity, ISBN 0-201-38423-X Hard-cover. Back cover: "Spacetime tells matter how to move; mass tells spacetime how to curve."
- ^ H.D. Young & R.A. Freedman, University Physics with Modern Physics: 11th Edition: International Edition (2004), Addison Wesley. Chapter 1, section 1.1, page 2 has this to say: "Physics is an experimental science. Physicists observe the phenomena of nature and try to find patterns and principles that relate these phenomena. These patterns are called physical theories or, when they are well established and of broad use, physical laws or principles."
- ^ Steve Holzner, Physics for Dummies (2006), Wiley. Chapter 1, page 7 says: "Physics is the study of your world and the world and universe around you." See Amazon Online Reader: Physics For Dummies (For Dummies(Math & Science)), last viewed 24 Nov 2006.
- ^ Evidence exists that the earliest civilizations dating back to beyond 3000 BC, such as the Sumerians, Ancient Egyptians, and the Indus Valley Civilization, all had a predictive knowledge and a basic understanding of the motions of the Sun, Moon, and stars.
- ^ Alhazen's Book of Optics (1021) and Francis Bacon's Novum Organum (1620) were critical in the development of scientific method.
- ^ Change in velocity of light causes apparent changes in mass,dimension and time. Taylor, Edwin F. & Wheeler, John Archibald (1966), Spacetime Physics, San Francisco: W.H. Freeman and Company, ISBN 0-7167-0336-X See, for example, The Relativistic Rocket, Problem #58, page 141, and its worked answer.
- ^ Einstein, Albert (November 25, 1915). "Die Feldgleichungen der Gravitation". Sitzungsberichte der Preussischen Akademie der Wissenschaften zu Berlin: 844–847. Retrieved on 2006-09-12.
- ^ Einstein, Albert (1916). "The Foundation of the General Theory of Relativity" (PDF). Annalen der Physik. Retrieved on 2006-09-03.
- ^ Perrot, Pierre (1998). A to Z of Thermodynamics. Oxford University Press. ISBN 0-19-856552-6.
- ^ Clark, John, O.E. (2004). The Essential Dictionary of Science. Barnes & Noble Books. ISBN 0-7607-4616-8.
- ^ Clausius, Ruldolf (1850). On the Motive Power of Heat, and on the Laws which can be deduced from it for the Theory of Heat. Poggendorff's Annalen der Physick, LXXIX (Dover Reprint). ISBN 0-486-59065-8.
- ^ Van Ness, H.C. (1969). Understanding Thermodynamics. Dover Publications, Inc.. ISBN 0-486-63277-6.
- ^ Dugdale, J.S. (1998). Entropy and its Physical Meaning. Taylor and Francis. ISBN 0-7484-0569-0.
- ^ Max Planck (1925), A Survey of Physical Theory derives his law of blackbody radiation in the notes on pp. 115-116, ISBN 0-486-67867-9
- ^ Feynman Lectures on Physics, vol I p. 41-6, ISBN 0-201-02010-6
- ^ See, for example, the influence of Kant and Ritter on Oersted.
- ^ Yet, universalism is encouraged in the culture of physics. For example, the World Wide Web, which was innovated at CERN by Tim Berners-Lee, was created in service to the computer infrastructure of CERN, and was/is intended for use by physicists worldwide. The same might be said for arXiv.org
- ^ "Division of Condensed Matter Physics Governance History". Retrieved on 2007-02-13.
- ^ Stanford Applied Physics Department Description
[edit] Further reading
Real World Physics
A description of the physical principle behind doppler radar.
Categories
- Optics
- Laser physics
- Quantum optics and information
- Structure and dynamics of atoms, molecules and clusters
- Collision dynamics
- Laser-matter interactions
- Fundamental constants and physical laws
- Optical materials and metamaterials
- Optical traps and optical lattices
- Plasma production
- Plasma confinement
- Plasma theory and modelling
- Fusion reactor engineering and technology
- Fusion reactor theory
- Plasma astronomy
- Plasma technology
- Elementary particles and fields (experimental)
- Elementary particles and fields (theoretical)
- Nuclear physics (experimental)
- Nuclear physics (theoretical)
- Particle astrophysics (experimental)
- Particle astrophysics (theoretical)
- Nuclear reactor physics
- Nanostructures and atomic-scale physics
- Surfaces
- Interfaces
- Liquids
- Magnetism
- Semiconductors
- Superconductivity
- Electronic properties
- Low-temperature physics
- Early universe
- Dark matter and dark energy
- Gravitational physics
- Particle and nuclear astrophysics
- String theory and cosmology
- Large-scale structures
- Stellar formation and evolution
- Solar physics
- Planetary physics
- Space observation and exploration
- Chaotic and complex systems
- Exact results
- Quantum mechanics
- Statistical mechanics
- Field theory
- Critical phenomena
- Highly correlated systems
- Non-equilibrium processes
- Computational biology
- Applied mathematics
- Sensors
- Metrology
- Imaging
- Microscopy
- Data acquisition and analysis
- Vacuum systems
- Nuclear medicine
- Imaging
- Biophysics
- Geodynamics and geomagnetism
- Seismology
- Geological exploration
- Biodiversity
- Atmospheric physics/climate change
- Energy
Welcome to Physics 2000, an interactive journey through modern physics! Have fun learning visually and conceptually about 20th Century science and high-tech devices.
What is it?
Find out more about us, how to use this site, and meet our cast of characters.Einstein's Legacy
Explore how Einstein's revolution in physics has led to X-rays, microwave ovens, lasers, and many other modern devices. Click here to see applets from this unit.The Atomic Lab
See how some surprising 20th Century physics experiments show us that not everything is as it seems... Click here to see applets from this unit.Science Trek
Go where few ever go - into the mind of the theoretical scientist! Learn basic principles of waves, quantum mechanics, polarization, and the periodic table. Click here to see applets from this unit.
This Month in Physics History
July, 1977: MRI Uses Fundamental Physics for Clinical Diagnosis
![]() |
I. I. Rabi |
On July 3, 1977, the first magnetic resonance imaging (MRI) exam on a live human patient was performed. MRI, which identifies atoms by how they behave in a magnetic field, has become an extremely useful non-invasive method for imagining internal bodily structures and diagnosing disease. The life-saving medical technique has its foundations in the work of physicist I. I. Rabi, who during the 1930s developed a method of measuring magnetic properties of atomic nuclei.
Isidor Isaac Rabi was born on July 29, 1898 in Rymanow, Austria. In 1899 his family moved to New York, where they lived in poverty in the Lower East Side before moving to Brooklyn in 1907. Rabi’s parents were Orthodox Jews, and though Rabi never practiced religion as an adult, he was always influenced by his religious upbringing. He felt that doing good physics was “walking the path of God.”
Rabi graduated from Cornell University in 1919 with a degree in chemistry. But he wasn’t really captivated by chemistry, and spent three years not doing much of anything before deciding to go to graduate school in physics at Cornell. After finishing his PhD in 1927, Rabi went to Europe, where he spent time working with the giants of quantum mechanics, including Sommerfeld, Bohr, Pauli, Stern, and Heisenberg.
Rabi was fascinated by quantum ideas, especially the Stern–Gerlach experiment. Otto Stern and Walther Gerlach had sent a thin beam of silver atoms through a non-uniform strong magnetic field, and observed that the beam separated into two distinct sub-beams, the atoms in the beam having been deflected slightly according to the direction of their magnetic moments.
When Rabi returned to the United States in 1929, he took a teaching position at Columbia University. After spending two years searching for a problem that interested him, in 1931 Rabi set up his molecular beam lab and took up the problem of determining the nuclear spin and associated magnetic moment of sodium. The nuclear magnetic moment, much smaller than that of the electron, was difficult to determine precisely. Rabi and Gregory Breit figured out how to modify the classic Stern-Gerlach apparatus to find the nuclear spin of sodium.
Rabi, who was often viewed as lazy, was always impatient with routine experimental techniques and data analysis. He liked to say he wanted an answer at the end of the day, and was driven to design clever, clean experimental methods, methods that brought him “nearer to God.”
Throughout the 1930s, Rabi improved the molecular beam method and used it to gather increasingly accurate values for the nuclear spin of atoms, including hydrogen and deuterium. The work culminated in the magnetic resonance method which is the basis for magnetic resonance imaging.
Magnetic moments tend to align either parallel or antiparallel to an external magnetic field, and tend to behave somewhat like tops, precessing about the direction of the magnetic field, with a frequency that depends on the magnetic field strength and the atom’s nuclear magnetic moment. In 1937 Rabi predicted that the magnetic moments of nuclei in these experiments could be induced to flip their orientation if they absorbed energy from an electromagnetic wave of the right frequency. They would also emit this amount of energy in falling back to the lower energy orientation. Rabi would be able to detect this transition from one state to the other. He called his method molecular beam magnetic resonance.
![]() |
MRI Image |
Rabi and his team modified the molecular beam apparatus so the beam was also exposed to a radio frequency signal as it traveled through the magnetic field. Tuning either the external magnetic field or the radio frequency can produce resonance. They observed the first magnetic resonance absorption in 1938, with beam of lithium chloride molecules. Rabi was enthralled by the flopping of the magnetic moment, and the group held a party to celebrate the achievement.
Each atom or molecule has a characteristic pattern of resonance frequencies. Rabi detected a series of resonances in different molecules that could be used to identify the type of atom or molecule and give more detail into molecular structure.
After World War II broke out, Rabi left his molecular beam laboratory and went on to become Associate Director of the MIT Radiation Laboratory. He was awarded the Nobel Prize in 1944, “for his resonance method for recording the magnetic properties of atomic nuclei.”
In 1946 Edward Purcell and Felix Bloch independently found a way to study the magnetic resonance properties of atoms and molecules in solids and liquids, instead of individual atoms or molecules as in Rabi’s molecular beam method. Later, nuclear magnetic resonance was further developed into the imaging technique that is now commonly used for medical diagnosis. The first images were produced in the early 1970s, and the first live human subject was imaged in 1977. MRI machines became commercially available in the 1980s, and are now commonly used for imaging internal body structures, especially soft tissues like the brain.
Shortly before he died in January of 1988, Rabi was imaged in an MRI machine. “It was eerie. I saw myself in that machine,” he said. “I never thought my work would come to this.”
Physics is the science of matter and how matter interacts.
Contents[hide] |
[change] What is physics?
Physics is the study of matter and energy in space and time and how they are related to each other. Physicists assume (take as given) the existence of mass, length, time, electric charge and temperature and then define (give the meaning of) all other physical quantities in terms of these basic units. Mass, length, time, electric charge and temperature are never defined but the standard units used to measure them are always defined. In the Mks system, the meter is the basic unit of length, the kilogram is the basic unit of mass, the second is the basic unit of time, the coulomb is the basic unit of electric charge. Temperature may be expressed in degree Celsius, degree Fahrenheit, or in degree Kelvin. The International System of Units (abbreviated SI from the French Système International) is the world's most widely used system, both in everyday commerce and in science but the United States has not adopted it yet.
Physics studies how things move, and the forces that make them move. For example, velocity and acceleration are used by physics to show how things move. Also, physicists study the forces of gravity, electricity, magnetism and the forces that hold matter together.
Physics studies very large things, and very small things. For example, physics studies stars, planets and galaxies and other big pieces of matter. Physics also studies small pieces of matter, such as atoms and electrons.
Physics also studies sound, light and other waves. Physics studies energy, heat and radioactivity, and even space and time. Physics not only helps people understand how objects move, but how they change form, how they make noise, how hot or cold they will be, and what they are made of at the smallest level.
[change] Physics uses numbers
Physics is a quantitative science because it is based on numerical measurements. Mathematics is used in physics to make models and predictions of how nature behaves. The predictions are compared to the way the real world works. Physicists are always working to make their models of the world better.
[change] Less simple
[change] General description
Physics is the science of matter and how matter interacts. Physics is used to describe the physical universe around us, and to predict how it will behave. Physics is the science concerned with the discovery and characterization of the universal laws which govern matter, movement and forces, and space and time, and other features of the natural world.
[change] Breadth and goals of physics
The sweep of physics is broad, from the tiniest components of matter and the forces that hold it together, to galaxies and even larger structures. There are only four forces that appear to operate over this entire range. However, even these four forces (gravity, electromagnetism, the weak force associated with radioactivity, and the strong force which holds atoms together) are believed to be different aspects of a single force.
Physics is primarily focused on the goal of formulating ever simpler, more general, and more accurate rules that govern the character and behavior of matter and space itself. One of the major goals of physics is the formulation of theories of universal applicability. Therefore, physics can be viewed as the study of those universal laws which define, at the most fundamental level possible, the behavior of the physical universe.
[change] Physics uses the scientific method
Physics uses the scientific method. That is, data from experiments and observations are collected. Theories which attempt to explain these data are produced. Physics uses these theories to not only describe physical phenomena, but to model physical systems and predict how these physical systems will behave. Physicists then compare these predictions to observations or experimental evidence to show whether the theory is right or wrong.
The theories that are well supported by data and are especially simple and general are sometimes called scientific laws. Of course, all theories, including those known as laws, can be replaced by more accurate and more general laws, when a disagreement with data is found.[1]
[change] Physics is Quantitative
Physics is more quantitative than most other sciences. That is, many of the observations in physics may be represented in the form of numerical measurements. Most of the theories in physics use mathematics to express their principles. Most of the predictions from these theories are numerical. This is because of the areas which physics has addressed are more amenable to quantitative approaches than other areas. Sciences also tend to become more quantitative with time as they become more highly developed, and physics is one of the older sciences.
[change] Fields of physics
Classical physics traditionally included the fields of mechanics, optics, electricity, magnetism, acoustics and thermodynamics. Modern physics is a term normally used to cover fields which rely on quantum theory, including quantum mechanics, atomic physics, nuclear physics, particle physics and condensed matter physics, as well as the more modern fields of general and special relativity. Although this distinction can be commonly found in older writings, it is of limited current significance as quantum effects are now understood to be of importance even in fields previously considered purely classical.[2]
[change] Approaches in physics
There are many approaches to studying physics, and many different kinds of actitivies in physics. There are two main types of activities in physics; the collection of data and the development of theories.
The data in some subfields of physics is amenable to experiment. For example, condensed matter physics and nuclear physics benefit from the ability to perform experiments. Experimental physics focuses mainly on an empirical approach. Sometimes experiments are done to explore nature, and in other cases experiments are performed to produce data to compare with the predictions of theories.
Some other fields in physics like astrophysics and geophysics are primarily observational sciences because most their data has to be collected passively instead of through experimentation. Nevertheless, observational programs in these fields uses many of the same tools and technology that are used in the experimental subfields of physics.
Theoretical physics often uses quantitative approaches to develop the theories that attempt to explain the data. In this way, theoretical physics often relies heavily on tools from mathematics. Theoretical physics often can involve creating quantitative predictions of physical theories, and comparing these predictions quantitatively with data. Theoretical physics sometimes creates models of physical systems before data is available to test and validate these models.
These two main activities in physics, data collection and theory production and testing, draw on many different skills. This has led to a lot of specialization in physics, and the introduction, development and use of tools from other fields. For example, theoretical physicists apply mathematics and numerical analysis and statistics and probability and computers and computer software in their work. Experimental physicists develop instruments and techniques for collecting data, drawing on engineering and computer technology and many other fields of technology. Often the tools from these other areas are not quite appropriate for the needs of physics, and need to be adapted or more advanced versions have to be produced.
[change] Physicists
There are many famous physicists. Isaac Newton studied gravity. Galileo Galilei studied light and how planets move. Albert Einstein made a theory for how light can make electrons move, and studied how gravity affects light and space. Heinrich Hertz discovered that light is a type of electromagnetic wave.
Ernest Rutherford said that "Physics is the only real science. The rest are just stamp collecting."
[change] See also
- Physics links
- Astronomy
- Electronics
- Engineering
Microsoft Games to Use Havok's Software Physics
Microsoft has inked a long-term deal with Havok, allowing all of their internal and affiliated studios full access to the company's entire library of physics tools.
This perpetual licensing agreement is expected to reduce both development time and costs across the board for any Microsoft partners choosing to take advantage of Havok's offerings.
Havok will be providing Microsoft with their Havok Physics, Animation, and Behavior suites, in addition to any Havok products that crop up in the future -- the recently unveiled Havok Cloth and Destruction, for instance.
Already practically synonymous with digital physics, Havok has had a hand in Microsoft products past, present, and future, including everything from Halo Wars to Scene It? Lights, Camera, Action. Havok managing director David O'Meara said the following regarding their arrangement with Microsoft:
"Havok is committed to providing not only the premier physics software to our customers, but also in giving them new tools, such as Havok Behavior, that can substantially reduce the time it takes teams to develop character behaviors, allowing game programmers to focus on developing the best game they can. Microsoft has been a valuable customer of ours for seven years and we are excited about the development teams having access to the entire suite of current Havok products, plus our upcoming products Havok Cloth and Havok Destruction."
While this may not sound terribly exciting to your average frat guy plugging away at his ninth prestige level in Call of Duty 4, this deal should ultimately translate to Microsoft developers being able to focus more on getting their games in your hands.
Hackers Beware - New Technique Uses Photons, Physics To Foil Codebreakers
ScienceDaily (Feb. 23, 2006) — For governments and corporations in the business of transmitting sensitive data such as banking records or personal information over fibre optic cables, a new system demonstrated by University of Toronto researchers offers the protective equivalent of a fire-breathing dragon.
See also:“Quantum cryptography is trying to make all transmissions secure, so this could be very useful for online banking, for example,” says Professor Hoi-Kwong Lo, an expert in physics and electrical and computer engineering at U of T’s Centre for Quantum Information and Quantum Control and the senior author of a new study about the technique. “The idea can be implemented now, because we actually did the experiment with a commercial device.”
The study describes the first experimental proof of a quantum decoy technique to encrypt data over fibre optic cable. In quantum cryptography, laser light particles (photons) carry complex encryption keys through fibre optic cables, dramatically increasing the security of transmitted data. Conventional encryption is based on the assumed complexity of mathematical problems that traditional computers can solve. But quantum cryptography is based on fundamental laws of physics — specifically, Heisenberg’s Uncertainty Principle, which tells us that merely observing a quantum object alters it.
The technique varies the intensity of photons and introduces photonic “decoys,” which were transmitted over a 15-kilometre telecommunication fibre. After the signals are sent, a second broadcast tells the receiving computer which photons carried the signal and which were decoys. If a hacker tries to “eavesdrop” on the data stream to figure out the encryption key, the mere act of eavesdropping changes the decoys — a clear sign to the receiving computer that the data has been tampered with.
The study appears in the Feb. 24 issue of Physical Review Letters and was funded by Connaught, the Natural Sciences and Engineering Research Council of Canada, the Canada Research Chairs program, the Canada Foundation for Innovation, id Quantique, the Ontario Innovation Trust, a Premier’s Research Excellence Award, the Canadian Institute for Photonics Innovations, the Canadian Institute for Advanced Research and the University of Toronto.
Jülich Research Centre
Forschungszentrum Jülich GmbH (Jülich Research Centre Ltd.) is a member of the Helmholtz-Gemeinschaft or Hermann von Helmholtz Association of National Research Centres and is one of the largest interdisciplinary research centres in Europe. It was founded on 11 December 1956 by the Federal state of North Rhine-Westphalia as a registered association, before it became "Kernforschungsanlage Jülich GmbH" or Nuclear Research Centre Jülich in 1967. In 1990, the name of the association was changed to "Forschungszentrum Jülich GmbH".
[edit] Location
The Research Centre is situated in the middle of the Stetternich Forest in Jülich (Kreis Düren, Rheinland) and covers an area of 2.2 square kilometres.
[edit] Financing
The annual budget of the Research Centre is approximately € 360 million. Public funds are split between the German Federal Government (90 %) and the Federal State of North Rhine-Westphalia (10 %).
[edit] Staff/size
The Research Centre employs more than 4,300 members of staff (2006) and works within the framework of the disciplines physics, chemistry, biology, medicine and engineering on the basic principles and applications in the areas of health, information, environment and energy. Amongst the members of staff, there are approx. 1,300 scientists including 400 PhD students and 150 diploma students. Around 800 people work in the administration and service areas, 350 work for project management agencies, and there are 1,500 technical staff members, while around 350 trainees are completing their training in 20 different professions.
Some 700 visiting scientists come to Research Centre Jülich every year from more than 50 different countries.
[edit] Training and apprenticeships at Research Centre Jülich
In 2003, 367 people were trained in 20 different professions in the Research Centre. The proportion of trainees lies around 9 % and is more than twice as high as the German national average (for companies with more than 500 employees). In cooperation with RWTH Aachen University and Aachen University of Applied Sciences, the Research Centre also offers combined practical and academic courses. After they have successfully completed their exams, graduates are offered six months employment in their chosen profession. Between 1959 and 2007 around 3,800 trainees completed their training in more than 25 different professions.
No lectures are held at the Research Centre itself, but in line with the so-called "Jülich model", the directors of the institutes are appointed professors at nearby universities in a joint procedure with the Federal State of North Rhine-Westphalia (usually Aachen, Bonn, Cologne, Dusseldorf, but also universities farther away such as Duisburg-Essen or Münster). By holding a lectureship there, they can fulfil their teaching duties. Many other scientists at the Research Centre who have achieved habilitation also undertake lectureships in the nearby universities. In cooperation with the universities, what are known as "research schools" (e.g. "German Research School for Simulation Science" with RWTH Aachen University or "International Helmholtz Research School of Biophysics and Soft Matter" with the universities of Cologne and Dusseldorf) are founded in an effort to support the scientific training of students.
An exception to this is the training of mathematical-technical assistants. In cooperation with Aachen University of Applied Sciences (Campus Jülich), the lectures required for the B.Sc. in "Scientific Programming" are largely held in the Central Institute for Applied Mathematics (ZAM) by university professors and ZAM instructors. For the subsequent M.Sc. in "Technomathematics", the same model applies and some of the lectures are held by ZAM staff.
Every year, Research Centre Jülich hosts a two-week IFF Summer School, which addresses current issues in solid-state physics.
[edit] Structure
[edit] Organisation
The Research Centre is organised into
- 7 institutes,
- 4 central divisions,
- 2 programme groups,
- 2 projects and
- 2 project management organizations
- Project Management Jülich
- Project Management Organization "Energy, Technology, Sustainability" (ETN)
[edit] Bodies
The bodies of the Research Centre are
- the Partners' Meeting
- the Supervisory Board
- the Board of Directors, which is made up of
- Prof. Dr. Achim Bachem (Chairman)
- Dr. Ulrich Krafft (Deputy Chairman), and
- the Scientific and Technical Council (WTR)
[edit] Research at Research Centre Jülich
Research at Jülich is divided into four research areas: health, information, environment, and energy. The key competencies of physics and scientific computing provide the basis for world-class research in these areas.[1] The various institutes and project groups are categorised under five research areas, each of which is headed by a research director:
- Health: (Research Director: Prof. Karl Zilles)
- Institute of Neurosciences and Biophysics
- Cellular Biophysics (INB-1)
- Molecular Biophysics (INB-2)
- Medicine (INB-3)
- Nuclear Chemistry (INB-4)
- Institute of Biotechnology (IBT)
- Institute of Neurosciences and Biophysics
- Information: (Research Director: Prof. H. Müller-Krumbhaar)
- Institute of Bio- and Nanosystems (IBN)
- Institute of Solid State Research (IFF)
- Environment (Research Director: Prof. U. Schurr)
- Institute of Chemistry and Dynamics of the Geosphere (ICG)
- Stratosphere (ICG-1)
- Troposphere (ICG-2)
- Phytosphere (ICG-3)
- Agrosphere (ICG-4)
- Sedimentary Systems (ICG-5)
- Institute of Chemistry and Dynamics of the Geosphere (ICG)
- Energy: (Research Director: Detlev Stöver)
- Institute of Energy Research (IEF)
- Materials Synthesis and Processing (IEF-1)
- Microstructure and Properties of Materials (IEF-2)
- Fuel Cells (IEF-3)
- Plasma Physics (IEF-4)
- Photovoltaics (IEF-5)
- Safety Research and Reactor Technology (IEF-6)
- Systems Analysis and Technology Evaluation (IEF-STE)
- Fuel Cell Project (IEF-PBZ)
- Nuclear Fusion Project (IEF-KFS)
- Institute of Energy Research (IEF)
- Key Competencies: (Research Director: Prof. Hans Lüth)
- Scientific Computing:
- Physics:
- Institute of Solid State Research (IFF)
- Institute of Nuclear Physics (IKP)
- Jülich Centre for Neutron Science (JCNS)
- Ernst Ruska Centre for Microscopy and Spectroscopy with Electrons (ER-C)
[edit] Large-scale facilities at Research Centre Jülich
[edit] Cooler Synchrotron COSY
COSY (Cooler Synchrotron) is a particle accelerator (synchrotron) and storage ring (circumference: 184 m) for accelerating protons and deuterons operated by the Institute of Nuclear Physics (IKP) in the Research Centre.
COSY is characterised by what is known as beam cooling, which reduces the deviation of particles from their predetermined path (can also be understood as the thermal motion of particles) using electron or stochastic cooling. At COSY there are a number of experimental facilities for studies in the field of hadron physics. These include the ANKE magnetic spectrometer, the TOF flight spectrometer and the WASA universal detector, which was moved to COSY from the CELSIUS storage ring of The Svedberg Labor (TSL) in Uppsala in 2005.
COSY is one of the only accelerators in the medium energy range with both electron cooling and stochastic cooling.
The synchrotron is used by scientists from German and foreign research institutions at internal and external target stations. It is one of the research facilities used for collaborative research supported by the Federal Ministry of Education and Research (Germany).
[edit] Research reactor FRJ-2
FRJ-2 is a reactor of the same class as DIDO and is used for neutron scattering experiments. It is operated by the Central Research Reactors Division (ZFR). FRJ-2 is the strongest neutron source in the Helmholtz Association and it is primarily used to conduct scattering and spectroscopic experiments on condensed matter. On May 2, 2006, FRJ-2 was shut down after almost 44 years or 18,875 days of operation. The experiments at FRJ-2 were dismantled bit by bit and transferred to the FRM-II research reactor in Munich.
[edit] Supercomputers
The following supercomputers are all operated in Jülich by the Central Institute for Applied Mathematics (ZAM) within the framework of the John von Neumann Institute for Computing (NIC).
[edit] JUGENE
From autumn 2007 the JUGENE, an IBM Blue Gene/P computer is running and was officially started in February 2008. Its 65,000 processors should reach 220 TFLOPS. It is the fastest computer in Europe and the second fastest in the world.[2]
[edit] JUBL
On March 6, 2006, a massively parallel supercomputer, based on IBMs Blue Gene/L architecture and known as JUBL, Jülich BlueGene/L Super Computer, was put into operation.
With 16,384 processors (8192 nodes each with two processors) and an internal memory of 4.1 terabytes (512 megabytes per node), the computer is capable of a peak performance (Rpeak) of 45.87 TFLOPS. The LINPACK performance (Rmax) is 37.33 TFLOPS. At the time when it officially went into operation, JUBL was the 6th most powerful computer in the world.
[edit] JUICE
Since spring 2007, JUICE (Juelich Initiative Cell Cluster) has been in operation. It is a cluster based on IBMs Cell microprocessor. Twelve QS20 blades with 24 Cell CPUs and 12GB RAM provide a peak LINPACK performance of 4.8 TFLOP/s. The cluster uses Mellanox 4x Infiniband cards and a 24-port Voltaire switch for highspeed communication.
[edit] IBM p690 Cluster Jump
The massively parallel supercomputer IBM p690 Cluster Jump has been in operation since the beginning of 2004.
With 1312 processors (41 nodes each with 32 processors) and an internal memory of 5 terabytes (128 gigabytes per node), the computer can achieve a maximum performance of 5.6 TFLOPS, which placed it at number 30 in the list of the most powerful computers in the world at the time of its inauguration. The nodes are linked to each other through a high performance switch (HPS). Through a globally parallel data system, applications have access to more than 60 terabytes of storage space and an integrated cassette storage with a capacity of one petabyte. The IBM p690 Cluster Jump is run on the AIX 5.1 operating system .
A new building (1,000 m²) was built especially for the IBM p690 Cluster Jump beside the Central Institute for Applied Mathematics.
[edit] CRAY SV1ex
No longer in operation
The vector computer CRAY SV1ex was the successor of CRAY J90, which was in operation between 1996 and 2002. It represented the next stage in the computer series of the parallel vector computers with a shared memory, CRAY X-MP, Y-MP and C90. With 16 CPUs and an internal memory of 32 gigabytes, the CRAY SV1ex had a performance of 32 GFLOPS. It was run on the UNICOS 10.0 operating system. This computer was decommissioned on June 30, 2005.
[edit] CRAY J90
No longer in operation
The vector computer CRAY J90 was used as a file server. It had 12 processors, an internal memory of 2 gigabytes and boasted a performance of 3 GFLOPS. CRAY J90 was also run on UNICOS 10.0 and it too was decommissioned on June 30, 2005.
[edit] TEXTOR tokamak
TEXTOR is a (Tokamak EXperiment for Technology Oriented Research) in the field of plasma-wall interaction operated by the Institute of Energy Research - Plasma Physics (IEF-4) in the Research Centre.
TEXTOR is used for research into nuclear fusion. In experiments, hydrogen is heated to a temperature of up to 50 megakelvins so that it takes the form of plasma. The interaction of this plasma with the surrounding walls is part of the research performed at the tokamak experiment. The knowledge gained will mainly be applied in the planned ITER fusion power plant, which is currently being constructed in Cadarache (South France) with the help of Research Centre Jülich.
[edit] 4 tesla magnetic resonance tomograph
A magnetic resonance tomograph (MRT) has also been in operation since 2004 in the Institute of Neurosciences and Biophysics - Medicine (INB-3). This tomograph has a magnetic field strength of 4 teslas. It is one of the most powerful devices in Germany and Europe. In addition, the two other tomographs (1.5 teslas and 3 teslas) are still used for functional imaging (fMRT), in particular, focusing on neurological, neuropsychological and psychiatric issues. In 2007, construction began on another 3.0-tesla tomograph with a PET application. Once the funding has been approved, a 9.4 tesla scanner combined with a PET will be constructed. Once this device has been built, it will be the most powerful MR tomograph in Europe (another tomograph with the same magnetic field strength already exists in the USA).
[edit] SAPHIR Atmosphere Simulation Chamber
In the 20-meter long SAPHIR chamber (Simulation of Atmospheric PHotochemistry In a large Reaction Chamber), a group in the Institute of Chemistry and Dynamics of the Geosphere - Troposphere (ICG-II) investigates photochemical reactions in the atmosphere.
[edit] PhyTec experimental facility for cultivating plants
Since 2003, a greenhouse with cutting-edge technology has been available at the Research Centre.[3] The maximum transparency of the panels (over 95 %) is achieved in the spectral range important for photosynthesis thanks to a special type of glass and an anti-reflective coating. Moreover, UV-B rays can pass through the glass panels. The CO2 concentration can be increased and decreased in two chambers, the humidity can be varied, and the temperatures can be kept at 25 °C, even in summer when the sun is constantly shining. Scientists at the Institute of Chemistry and Dynamics of the Geosphere - Phytosphere (ICG-III) simulate different climate scenarios here and investigate their influence on the key processes in plants, such as growth, transport, exchange processes with the atmosphere and soil, and biotic interactions.
[edit] Beamlines at synchrotrons
The Institute of Solid State Research (IFF) support a number of beamlines for research with synchrotron radiation at various synchrotrons:
- BL5 U-250-PGM at DELTA (Dortmund)
- UE56/1-SGM at BESSY (Berlin)
- MuCAT at APS (Argonne, USA)
- JUSIFA at HASYLAB (Hamburg)
[edit] Further research projects at Research Centre Jülich
[edit] CLaMS: Atmosphere Model for Climate Research
Understanding the chemical processes in the atmosphere is the basis of many climate models. Environmental researchers at Research Centre Jülich investigate the chemistry of the atmosphere with airplanes, balloons and satellites. They use their findings to generate chemical models such as CLaMS, which are then used in simulations on supercomputers.
[edit] MEM-BRAIN: carbon dioxide separation
Together with its research partners, Research Centre Jülich is developing ceramic membranes. These membranes could be put to use as filters in power plants, which would separate process gases and effectively retain carbon dioxide.[4]
[edit] UNICORE: easy access to computing resources
Today, computing and storage resources are often split between a number of computer systems, computer centres or even between different countries. Science and industry therefore need tools that will allow easy and secure access to these resources. UNICORE [1] from Jülich is one such grid-based software package.
[edit] Infrastructure
As well as research institutions and large-scale facilities, Research Centre Jülich has a variety of infrastructure units and central institutions that it needs for its day-to-day operations, including:
- Finance and Controlling Division (F)
- Personnel Division (P)
- Legal and Patent Division (R)
- Operation Management Division (B)
- Safety and Radiation Protection Division (S)
- Purchasing and Materials Division (M)
- Organization and Planning Division (O)
- Corporate Communications (UK)
- Central Institute for Applied Mathematics (ZAM)
- Central Technology Division (ZAT)
- Central Division of Analytical Chemistry (ZCH)
- Central Research Reactors Division (ZFR)
- Central Institute for Electronics (ZEL)
- Central Library (ZB)
[edit] See also
- AVR reactor
- In 2007, the Nobel Prize in Physics was awarded to Peter Grünberg and Albert Fert for the independent discovery of Giant Magnetoresistance. Grünberg was a leading researcher at the Jülich Research Centre.
[edit] External links
Refine Other Physical Measuring Instruments By
- China (mainland) (85)
- Singapore (3)
- India (2)
- United States (2)
- View all
- North America (82)
- South America (81)
- Western Europe (87)
- Eastern Europe (81)
- View all
- Manufacturer (92)
- Trading Company (42)
- Agent (15)
- Distributor/Wholesaler (18)
- View all
- OEM Service Offered (82)
- Design Service Offered (42)
- Buyer Label Offered (38)

GAUSS METER


China

China
QZJ automatic aerosol weight tester


China
Radio Frequency Card Prepaid Gas Meter


China

China
Electron Density-meter


China

China
Paper and Cardboard Thickness Tester


China
Intelligent Length Measurer


China

Hong Kong
Aerovane of the Wind Cup Style


China
paint thickness gauge


China
SYP1004-IV Engler Viscometer for Petroleum Products


China
Demonstrator of Metal Wire Expansion
phenomenon,...


China
Tempered Glass Stress Polarimeter Ldj-C


China

China

China

China
FUSIBILITY TESTER
FUSIBILITY TESTERFUSIBILITY TESTER
FUSIBILITY TESTER
FUSIBILITY TESTER...


China
Odometer Kamaz:5320-3802010


China
Grinder/polisher Mapao200M3/M5 with polishing head


China
Stormer Viscometer


China
Coating Thickness Gauge ED300


China
ETJ-4 DIGITAL THERMOMETER
Easy to read 31/2 LCD display
0.1 Degree Celsius accuacy and fast response...


China

China
Ultrasonic Thickness Gauge AR850


China
Laser Particle Size Analyzer (Dry Type Particle Analyzer)


China
Young's Modulus Measuring Instrument


China
No comments:
Post a Comment